The endeavor to control increasingly large systems of particles at the quantum level will be one of the driving forces for physical sciences in the coming decades. We aim to gain (i) deeper insight into complex dynamics that are influenced or even driven by quantum effects, and (ii) to control atoms and molecules at the highest level possible to set up many-body (model) systems, in a way, the ultimate form of engineering.
Our experimental work builds on different trapping technologies. On the one hand, on conventional rf-traps, while we extend this approach in size and dimension toward two-dimensional arrays of individual traps spanned by micro-fabricated surface-electrode traps. On the other hand, we aim to explore and exploit trapping of ions and atoms by optical means.
Fig. (left): Scaling Coulomb crystals in size and dimension while maintaining the unique controllability of the individual ion might allow addressing intriguing quantum effects in many different fields of physics. Here: Fluorescence image of 40 ions within one single trapping potential of our conventional radio-frequency (rf) trap, frozen by laser cooling into a 2D- to 3D Coulomb crystal.
Experimental Quantum Simulations
Direct experimental access to the most intriguing and puzzling quantum phenomena is extremely difficult and their numerical simulation on conventional computers can easily become computationally intractable. However, one might gain deeper insight into complex quantum dynamics via experimentally simulating and modeling the quantum behavior of interest in a second quantum system. There, the significant parameters and interactions might get precisely controlled, underlying quantum effects detected sufficiently well and their relevance could get revealed. This might remain valid for open systems, since the environment coupled to the simulator can be tailored with its impact being suspected to influence significantly the relevant quantum dynamics. Our group is dedicated to build such a model system based on trapped ions (and atoms), to derive and to perform two different classes of experimental quantum simulations: (1) addressing systems that might still be numerically tractable, however, hard or impossible to get realized in the laboratory. (2) addressing systems that remain numerically intractable, but where experimental results might assist in revealing underlying concepts. In the context of class (1), we proposed how to extend our experimental work on quantum walks on non orthogonal motional states, collaborating with the group of R. Werner (University of Hannover). In another approach, we discovered how to realize topologically protected defects within Coulomb crystals - in parallel to theoretical work done by B. Reznik and H. Landa (University of TelAviv) revealing the suitability of those defects to simulate solitons in the quantum regime.
Simulating discrete solitons
During the process of crystallization, a system will seek for perfect order (minimal energy). By evolving the phase transition too fast for communication between different sections of the crystal, sub-ensembles find perfect crystalline order, while becoming incommensurate at their borders. We demonstrated that these defects can oscillate as trapped quasi particles in their self-induced confining potential within the crystal. In close collaboration with theory, we reported about the properties of our experimental findings, such as, the different species of defects, their coupling to the mesoscopic environment of the crystal, their mutual (coherent) interaction and their potential prospects on accessing the quantum regime, provided by the unique, gapped structure of the related localized motional modes.
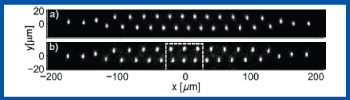
Fig. (right): 1D-2D Coulomb crystals. a) fluorescence image of ions in zigzag configuration. b) Only the ions out of the centre region (dashed box) form a zigzag structure as in (a), while the ions in the centre region are forming an extended topological defect: predicted to feature a discrete soliton.

Simulating quantum spin systems
The aim is not to copy the system and its dynamics in nature with its plethora of complex interactions. The aim is to reduce the system to its essential ingredients including the relevant impact by the environment and to test, in a controllable manner, the validity of theoretical assumptions and predictions. After our proof of principle experiment on quantum spin systems in the year 2008, following a proposal by Porras and Cirac, we further extended our simulator in size and dimensionality. Our efforts are two-fold: (i) Together with our collaborators from the Universities of Ulm, Madrid and Sussex, we further proposed how to extend the experimental toolbox, boosting the strength of interaction by using phonons directly for simulating particles exposed to artificial gauge fields and to photo-assist and control the dynamics of their tunneling between neighboring lattice sites simulated by the ions. We additionally study to exploit continuous sympathetic cooling of the phonon modes mediating relevant interaction in the model system, that is, permitting them to provide even more robust coherent (entangling) operations. The Fraunhofer Institute for Physical Measurement Techniques (IPM), funded by a private investor, is developing a novel laser concept and supporting us with a pilot system, especially designed for this purpose. (ii) Supported by our collaborators at the National Institute of Standards and Technology (NIST) and Sandia-National-Laboratories (SNL) we designed and realized a basic, two dimensional array of surface-electrode traps. In parallel, we tested a state-of-the-art liner trap that had been fabricated by SNL according to the identical (MEMS) technologies. We recently trapped ions within the fully functional triangular array.
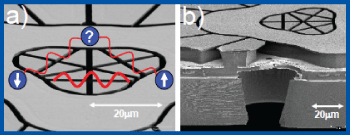
Fig. (above): Electron-microscopic images of our novel surface-electrode traps, a basic 2D array micro-fabricated by SNL. a) Bird’s eye view, the gaps isolating between rf and DC patches (ions and individually controllable interactions are animated). Three ions residing in three individual traps are suited to simulate anti-ferromagnetic interactions between three quantum spins. Classically, only two neighbouring spins can be orientated in an anti-ferromagnetic manner while the third spin becomes frustrated. Quantum mechanically, a superposition of all frustrated permutations, an entangled state, arises (in the envisioned, extended array, the whole ensemble of spins evolves adiabatically, via a quantum-phase-transition, into a tremendously complex state). b) Show case (similar as (a)), however, providing additional insight into the underlying structure, e.g., the electrodes’ extensions shielding the insulators and the cross section through a loading hole.
Trapping Ions in Atoms and Molecules Optically
Our group had achieved optical trapping of an ion in a dipole trap, recently followed by a 1D-optical lattice. There is a long-term prospect for scaling 2D quantum simulations in optical lattices, based on ions or even on ions and atoms. However, our current project of optically trapping an ion within a BEC of atoms is dedicated to overcome fundamental limitations set by micro-motion when combining optical and rf-trapping. We want to study how chemical reactions proceed at lowest temperatures? The classical concept predicts that approaching zero velocity is equivalent to a standstill of any dynamics. On the one hand, deviations can be expected, since the classical model is ceasing to be appropriate when particle-wave dualism is gaining importance. On the other hand, we might see quantum mechanical properties as minor corrections. However, in the regime of lowest temperatures quantum effects dominate and chemistry is predicted to obey fundamentally different rules. As a consequence, quantum chemistry might permit to control reactions and their pathways by external fields, since forces and related interactions become relevant compared to the kinetic energy. In collaboration with R. Mozynski (Univ. of Warsaw) we aim to study ultra-cold formation of BaRb+ within our optical traps. In a different context, however, developing the basic toolbox for operation, we performed a pump-probe experiment (5fs UV-pulses) on a single molecular ion. In collaboration with R. Cote (Univ. of Connecticut) we elaborate on options how to observe a BEC bound to an ion.
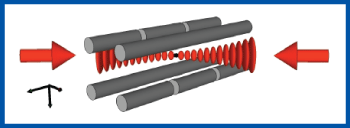